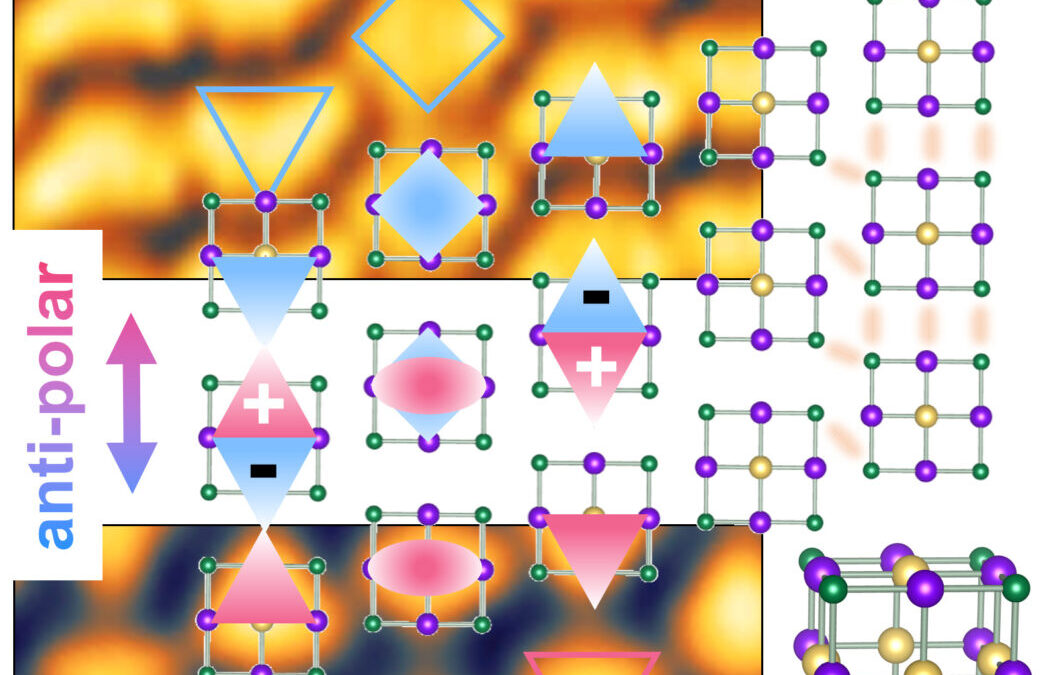
AuTeSe
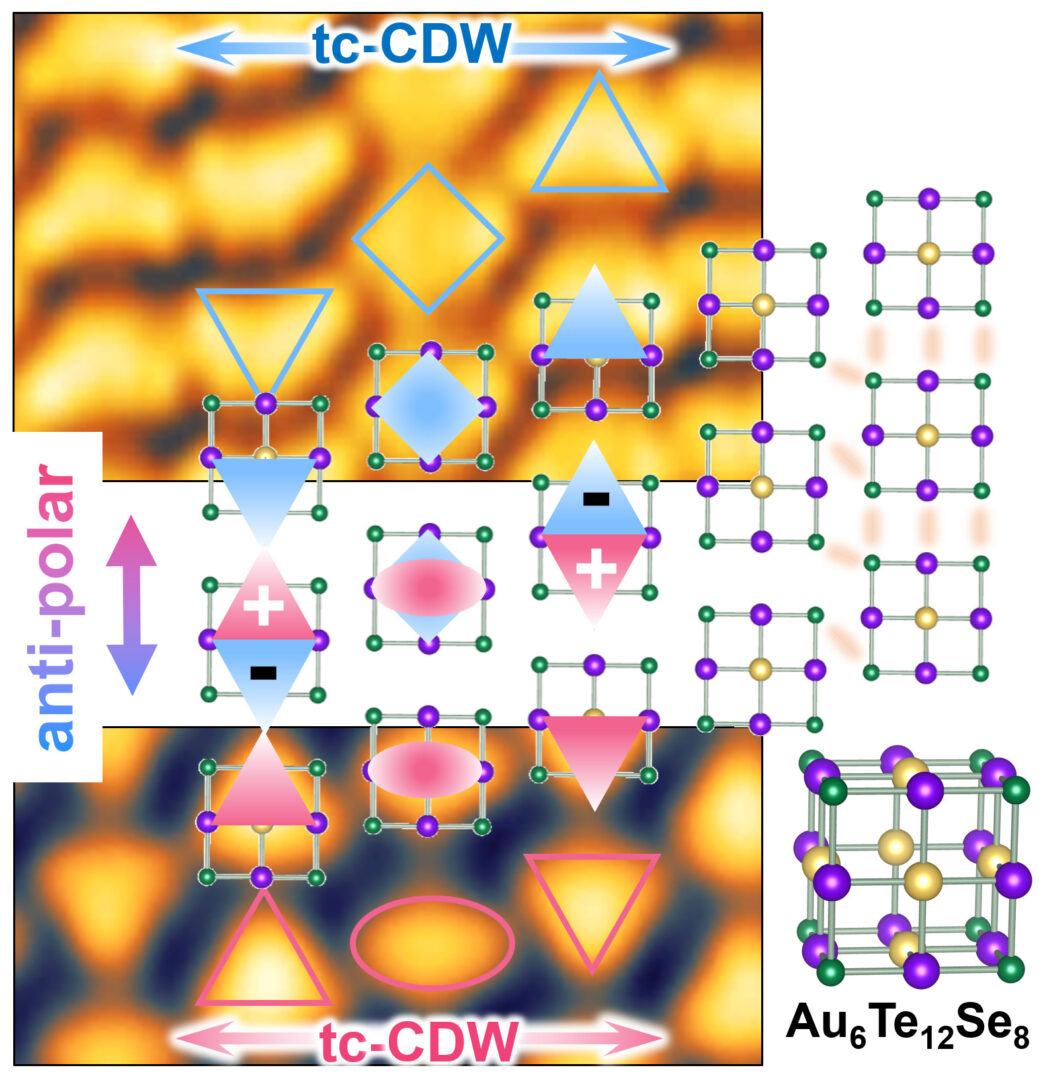
Fig.1 Intercube Te-Te quasibonds and two interweaved charge orders in the ATS superatomic crystal
.
Physical review X
Interweaving Polar Charge Orders in a Layered Metallic Super-atomic Crysta
A superatom is any cluster of atoms that collectively exhibits some properties of single atoms. When arranged into crystals through the noncovalent bonds, they can be readily assembled into nanostructures, because the reduced cohesive energy of the noncovalent bonds makes it easier to cleave the material. It is not yet clear whether such weakened energetic interaction is accompanied by a suppressed electronic interaction among the superatoms. To that end, we explore exotic electronic structures on the surface of one superatomic crystal and find strong electron-electron interactions do occur. We also find that two exotic charge orders emerge.
Recently, researchers synthesized a cubic superatom, Au6Te12Se8 (ATS), and assembled it into a 3D crystal with metallicity and superconductivity.[9] In our experiments, we observe two charge orders on the ATS surface. One is a charge density wave that forms across repeating columns of ATS cubes. The other is a polar metallic state that arises between the columns. The polar metallic states are of particular interest, suggesting the ATS surface is an antipolar metal—a type of exotic metal where metallicity and orderly, antiparallel-oriented electric dipoles coexist. The discovery of this antipoloar metal goes one step further toward the realization of multifunctional devices, which could, in principle, perform simultaneous electrical, magnetic, and optical functions. However, we have not yet examined ATS’s ferroelectricity, which is needed for electrical control of its electrical polarization.
This ATS crystal is, to the best of our knowledge, the first antipolar metal ever found and possesses the first polar metallic state hosted in superatomic units bound by noncovalent interactions. Thus, the strong electron-electron interactions, found in the 2D superatomic layers, open a category of quantum materials that contains versatile layered nanostructures exhibiting precisely tailorable electronic structures.
1. Z. Luo, A.W. Castleman, Special and General Superatoms, Accounts of Chemical Research 47 (2014) 2931-2940
2. Superatoms: Electronic and Geometric Effects on Reactivity, (2017)
3. E.A. Doud, A. Voevodin, T.J. Hochuli, A.M. Champsaur, C. Nuckolls, X. Roy, Superatoms in materials science, Nature Reviews Materials 5 (2020) 371-387
4. J. Puru, S. Qiang, Super Atomic Clusters: Design Rules and Potential for Building Blocks of Materials, Chemical Reviews 118 (2018) acs.chemrev.7b00524-
5. Z. Liu, X. Wang, J. Cai, H. Zhu, Room-Temperature Ordered Spin Structures in Cluster-Assembled Single V@Si12 Sheets, The Journal of Physical Chemistry C (2014)
6. E. Meirzadeh, A.M. Evans, M. Rezaee, M. Milich, C.J. Dionne, T.P. Darlington, S.T. Bao, A.K. Bartholomew, T. Handa, D.J. Rizzo, R.A. Wiscons, M. Reza, A. Zangiabadi, N. Fardian-Melamed, A.C. Crowther, P.J. Schuck, D.N. Basov, X. Zhu, A. Giri, P.E. Hopkins, P. Kim, M.L. Steigerwald, J. Yang, C. Nuckolls, X. Roy, A few-layer covalent network of fullerenes, Nature 613 (2023) 71-76
7. Evan, S., O’Brien, M., Tuan, Trin, Rose, L., Kann, Jia, Single-crystal-to-single-crystal intercalation of a low-bandgap superatomic crystal, Nature Chemistry (2017)
8. H. Yang, W. Yu, H. Huang, L. Gell, L. Lehtovaara, S. Malola, H. Hkkinen, N. Zheng, All-thiol-stabilized Ag44 and Au12Ag32 nanoparticles with single-crystal structures, Nature Publishing Group (2013)
9. Guo, J.G., Chen, X., Jia, X.Y. et al. Quasi-two-dimensional superconductivity from dimerization of atomically ordered AuTe2Se4/3 cubes. Nat Commun 8, 871 (2017)